Blog: Between the Frying Pan and the Fire – A critical look at Patient-Derived Organoids and Immortal Cell Lines: is it time to jump?
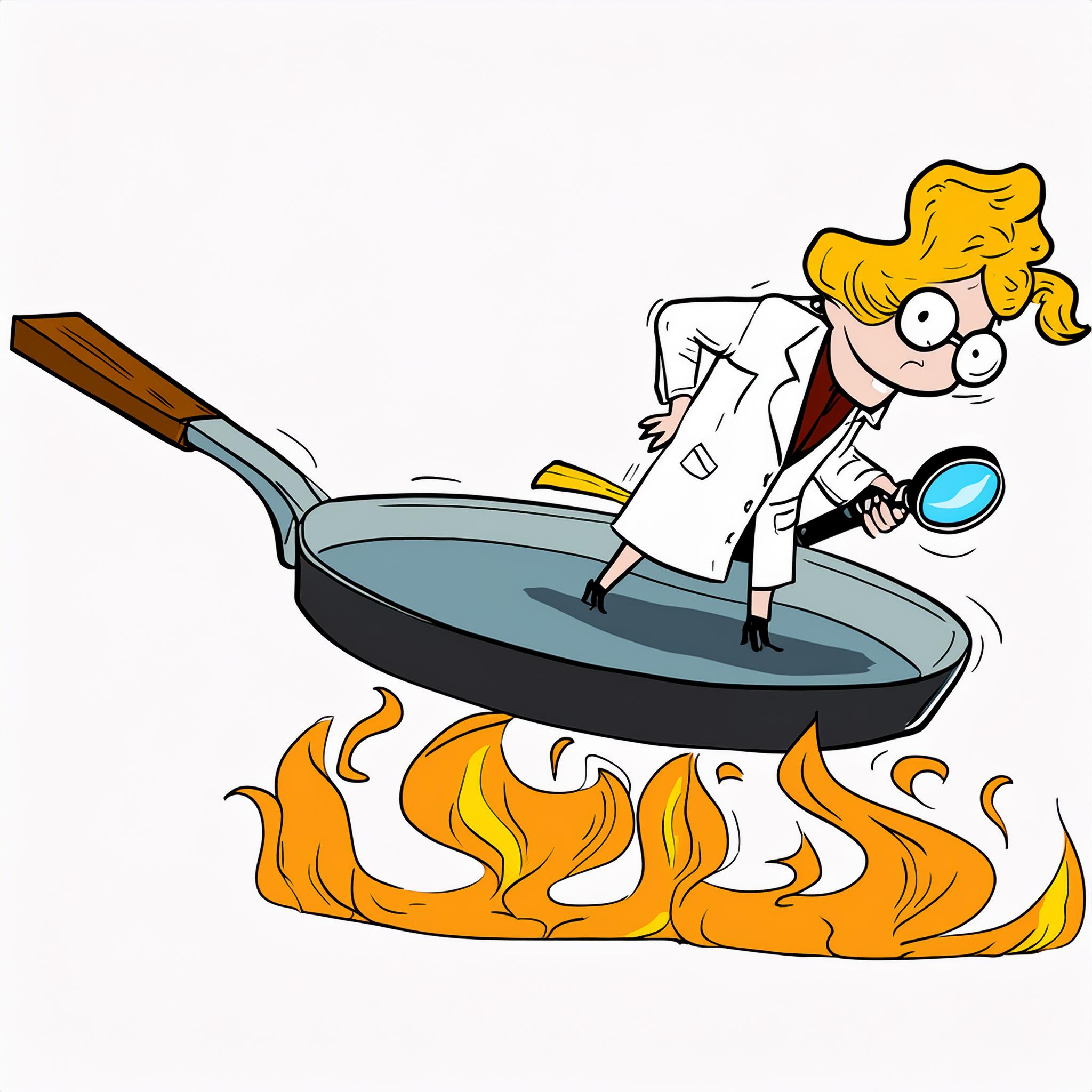
Cell lines are inexpensive, easy to handle and reproducible. For that reason they have been widely used for understanding the molecular basis of cancer and for drug screening. For example in 1990, the American National Cancer Institute selected a group of 60 different cell lines for these studies. However, over the ensuing years, data showed that, although retaining many characteristics of their parental tissue 1, these cell lines diverged from their parental cells with increasing passage numbers. They acquired differences in their DNA methylation pattern 2 and do not adequately reproduce drug sensitivities. Therefore after 25 years, during which more than 100,000 compounds were screened, this characteristic cohort of cell lines was retired 3. In its place, the NCI recommended developing thousands of patient derived xenografts (PDXs) by implanting small pieces of human tumours in immunosuppressed (‘nude’) mice. Although undoubtedly much more representative of human tumours, these new PDXs also suffer numerous limitations. For example, they are costly to develop; their behaviour depends on the site of implantation, and they are labour-intensive and expensive to maintain.
Cultural changes
Fortunately a number of simpler less expensive models have emerged. Researchers realised that the conventional methods to cultivate cells in flasks and plates were inadequate and induced the divergence from the parental cells in their tissue. From the 1950’s, the primary objective had been to successfully propagate cells in culture. Over the past 20 years, the focus shifted to growing cells in 3D ‘constructs’ in order to maintain (or recover) the cells’ in vivo physiological activities. In both tissues and tumours, cells experience diffusion gradients of gasses, nutrients and metabolites. In addition they are constantly communicating using a variety of channels. This shift in focus led to a realisation that cells need to be cultured as small 3D spheroids or organoids rather than flat 2D arrays, and led CelVivo to develop the ClinoStar and ClinoReactor products. Comparisons of the same cell line grown in the same environment but as 2D and 3D formats revealed a multitude of significant modifications in gene and protein expression.4,5 Culture as 3D spheroids allowed the HepG2/C3A cell line to predict toxicology better than primary hepatocytes for at least a few known drugs 6.
What are patient-derived organoids?
Patient-derived organoids are three-dimensional cell cultures derived from patient tissues or cells (PDCs) that mimic the complex cellular composition and architecture of the original tissue. They retain key characteristics and genetic features of the patient’s tumour or organ, providing a valuable tool for studying disease progression, drug responses, and personalized medicine approaches.
What are tumour-derived organoids?
Tumour-derived organoids (or tumouroids) are specific types of patient-derived organoids generated from tumour tissues. These organoids recapitulate the heterogeneity and cellular diversity of the original tumour, allowing for detailed investigation of tumour biology, drug screening, and therapeutic strategies, all tailored to individual patients.
The Advent of Patient Derived Organoids
Thus procedures were developed to cultivate either cell constructs, or small pieces of (minced) tissue (or tumour). These became known as patient derived spheroids and organoids (here collectively referred to as PDO). Their advantages are that they can, to varying degrees, recapitulate the complex architecture and functionality of human tissues, allowing function, disfunction and treatment to be studied in a more physiologically relevant environment 7,6.
PDOs have been shown to recapitulate numerous features characterising tumours in vivo including: histopathological subtypes, differentiation capacity and they can also mimic patient responses to drug treatment in the clinic.
Examples can be seen in the prostate 8; colorectal cancers 9; liver 10; pancreatic ductal adenocarcinoma 11; gastrointestinal 12; breast cancer 13.
Studies of the original tumour, PDO and PDX have shown that chromatin remodelling is driven by their respective microenvironments. 14
Individual patient cells can be imaged, assayed and even genetically edited (using CRISPR-Cas9 technologies) before and during PDO formation. Tumour subtypes illustrate an accumulation of multiple mutations or non-genetic changes that link tumour progression from the parental stem cell to independence. 15,11
A ‘Techtonic’ Shift at the FDA
These advantages have led to the FDA opening the door for testing compounds on 3D clusters of cells (like PDO), and no longer demanding animal testing. Even so this transition away from using animals for drug testing will still take many years due to numerous challenges. 16
As with Achilles, PDO’s have weaknesses that are inseparable from their strengths. The minced pieces of patient tissue contain variable populations of cells (patient derived cells PDCs) and differing extracellular matrices (ECM). Some (e.g. stem cell-PDCs) will out-compete others and over time this will lead to changes in the cell population and to the ECM composition. Furthermore, each cell type can undergo its own genetic drift. Another ‘weakness’ is that in vivo, cells functionality is plastic, allowing the cell to adapt to signals from other cells and to changes in their environment. These signals will change in vitro and thus, as with 2D culture, 3D culture will lead to functional divergence of the PDCs from the parental cells. Although it would be expected that this divergence will be more restricted and protracted compared to that seen in 2D cultures, no quantitative studies have yet been made to characterise this cultural drift.
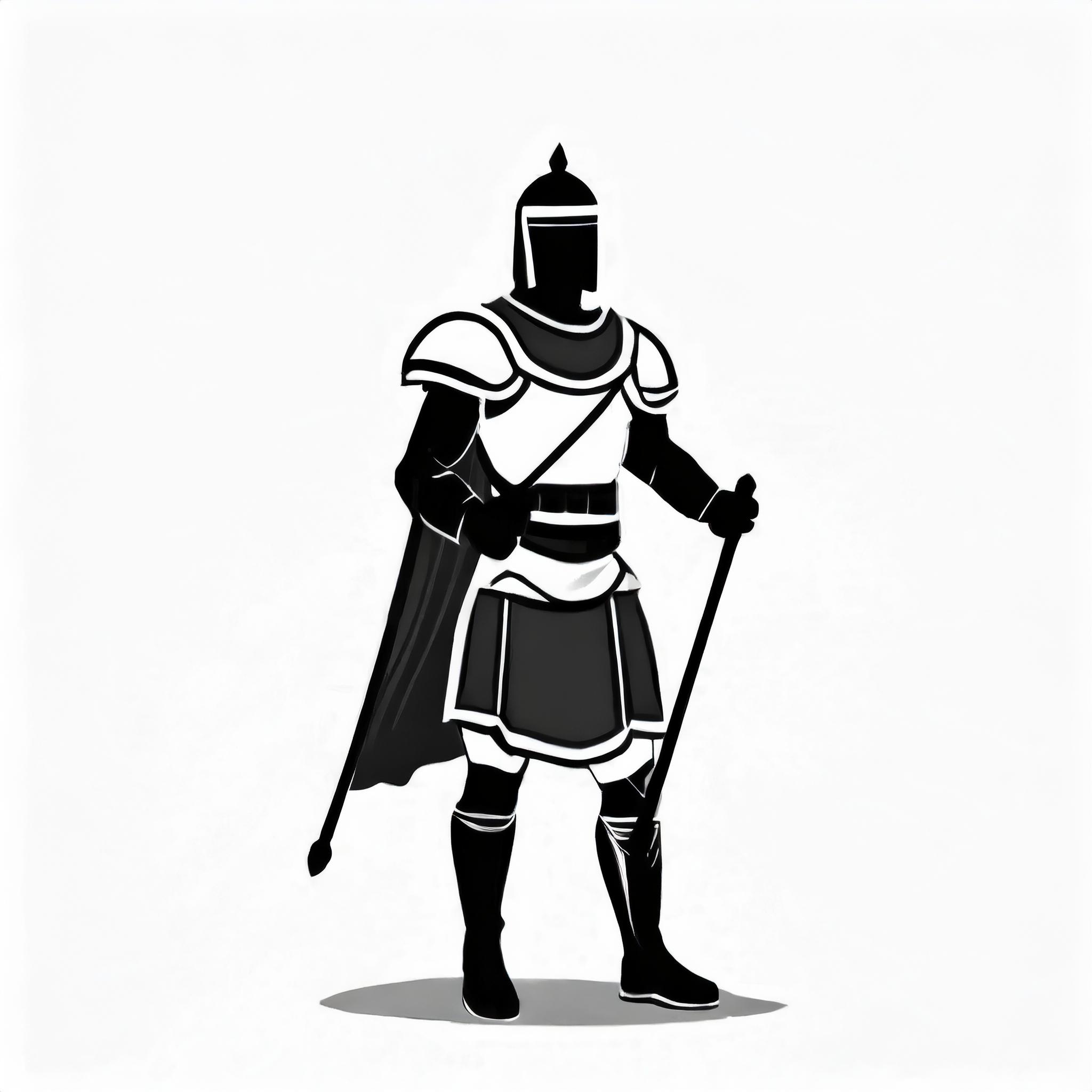
Thus to derive the maximal value of the data obtained, it makes sense to minimise the various types of stress that the PDO are exposed to. In vitro, PDO should be exposed to their native in vivo environment. Critical factors include the presence or absence of shear stress, diffusion gradients and appropriate gas tensions. They need to be shielded from reperfusion injury (sudden increases in oxygen tension and nutrient levels which can damage most cellular components). PDOs, depending on how dissociated the cells were, need to be given sufficient time to recover their in vivo structural integrity and phenotype (for example rebuild their cytoskeleton, ECM and communication pathways, readjust their metabolic balance and growth rate, reposition membrane pumps etc. all of which may take weeks).
Yet another Achilles weakness is that PDCs are mortal and may senesce. Thus while it is possible to expand the PDOs for personalising medicine or for high throughput screening, (spheroids 7) there is a finite limit.
Immortalisation of cells from patient derived organoids have been suggested as a solution to mortality and to make pdo cost effective. 17,18 In this way, with their accumulated mutations they begin to resemble cell lines (albeit one that is probably closer to the parental cell).
And this may bring us full circle! In the US, the ‘Toxicology in the 21st Century’ (Tox21) program is being developed by the Environmental Protection Agency (EPA), the Food and Drug Administration (FDA), the National Institutes of Health (NIH) and the National Toxicology Program (NTP) as partners. Their goal is to “rapidly and efficiently evaluate the safety of commercial chemicals, pesticides, food additives/contaminants, and medical products” (https://tox21.gov/overview/operational-model/). Interestingly, the majority of the assays used still employ cell lines (https://ncats.nih.gov/research/research-activities/Tox21/assays), including some old favourites (e.g. Hek293, HepG2 and HeLa) of the 60 which had been retired.
A strategy before we jump?
All in all, it boils down to a three point strategy.
- Define what question you want to answer
- Know the advantages and limitations of the system you are going to use
- Exclude extraneous factors that might influence your results.
Thus although before we jump out of the frying pan, we need to look carefully at the fire!
References
- Salvadores, M., Fuster-Tormo, F. & Supek, F. Matching cell lines with cancer type and subtype of origin via mutational, epigenomic, and transcriptomic patterns. Sci Adv 6 (2020).
- Varley, K.E. et al. Dynamic DNA methylation across diverse human cell lines and tissues. Genome Res 23, 555-567 (2013).
- Ledford, H. US cancer institute to overhaul tumour cell lines. Nature 530, 391 (2016).
- Wrzesinski, K. et al. The cultural divide: exponential growth in classical 2D and metabolic equilibrium in 3D environments. PLoS One 9, e106973 (2014).
- Wrzesinski, K. & Fey, S.J. Metabolic Reprogramming and the Recovery of Physiological Functionality in 3D Cultures in Micro-Bioreactors. Bioengineering (Basel) 5 (2018).
- Fey, S.J. & Wrzesinski, K. Determination of drug toxicity using 3D spheroids constructed from an immortal human hepatocyte cell line. Toxicol Sci 127, 403-411 (2012).
- Kunz-Schughart, L.A., Freyer, J.P., Hofstaedter, F. & Ebner, R. The use of 3-D cultures for high-throughput screening: the multicellular spheroid model. J Biomol Screen 9, 273-285 (2004).
- Pamarthy, S. & Sabaawy, H.E. Patient derived organoids in prostate cancer: improving therapeutic efficacy in precision medicine. Mol Cancer 20, 125 (2021).
- Barbachano, A. et al. Organoids and Colorectal Cancer. Cancers (Basel)
- Li, L. et al. Protein synthesis inhibitor omacetaxine is effective against hepatocellular carcinoma. JCI Insight 6 (2021).
- Seino, T. et al. Human Pancreatic Tumor Organoids Reveal Loss of Stem Cell Niche Factor Dependence during Disease Progression. Cell Stem Cell 22, 454-467 e456 (2018).
- Vlachogiannis, G. et al. Patient-derived organoids model treatment response of metastatic gastrointestinal cancers. Science 359, 920-926 (2018).
- Hogstrom, J.M. et al. Simultaneous isolation of hormone receptor-positive breast cancer organoids and fibroblasts reveals stroma-mediated resistance mechanisms. J Biol Chem 299, 105021 (2023).
- Xiang, K. et al. Chromatin Remodeling in Patient-Derived Colorectal Cancer Models. Adv Sci (Weinh), e2303379 (2024).
- Fujii, M. et al. A Colorectal Tumor Organoid Library Demonstrates Progressive Loss of Niche Factor Requirements during Tumorigenesis. Cell Stem Cell 18, 827-838 (2016).
- Wadman, M. FDA no longer needs to require animal tests before human drug trials. Science 379, 127-128 (2023).
- Rigaux, E. et al. Budget-Friendly Generation, Biochemical Analyses, and Lentiviral Transduction of Patient-Derived Colon Organoids. Curr Protoc 3, e943 (2023).
- Fey, C. et al. Enhancing pre-clinical research with simplified intestinal cell line models. J Tissue Eng 15, 20417314241228949 (2024).
Connecting Your Research to Reality
Learn more about how you can benefit from using the ClinoStar 2 and clinostat principle when culturing cells in your lab.
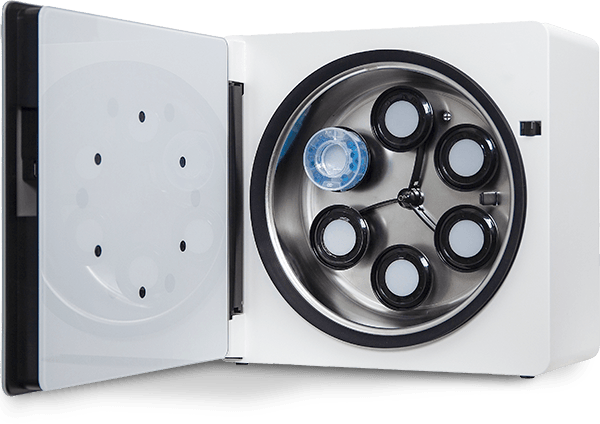