Blog: Unravelling the Dual Nature of Shear Stress in Cultured Cells
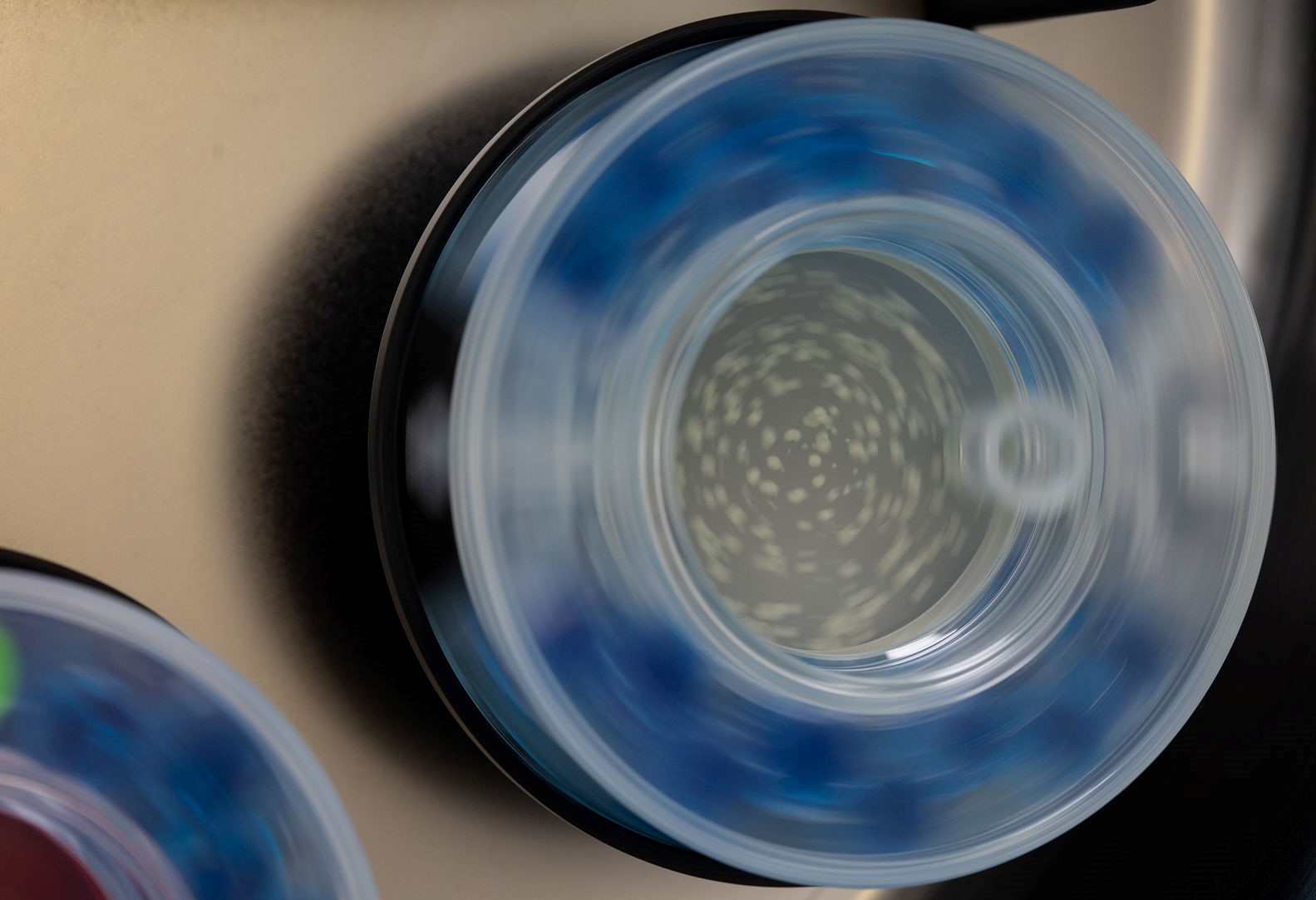
In cell culture, our expectation is that by exposing cells to conditions which mimic the in vivo environment from which they came, they will respond in a physiologically relevant manner.
When cells are grown as monolayers on flat surfaces, they have a direct access to oxygen. In this configuration an oxygen diffusion gradient builds up from the atmospheric oxygen source to its mitochondrial consumer and in due course, this will reach an equilibrium.
However, the number of cells will increase as they propagate. As the number of cell layers builds up in 3D constructs (e.g organoids or spheroids), the supply of oxygen down this diffusion gradient becomes more and more restrictive until it reaches a critical stage where oxygen becomes unavailable and the cell undergoes apoptosis.
In 3D cell culture, various strategies have been used to mitigate this outcome. They all involve reducing the length of the diffusion gradient, i.e. in effect bringing oxygenated media closer to the cell constructs. These strategies require mixing the media or flowing the media past the cells.
While this works well, it introduces a new factor into cell culture. The flow of media across the surface of the cell will introduce fluid flow shear stress (FSS).
Fluid Flow Shear Stress
FSS can trigger a number of different receptors to activate signal transduction pathways and change protein expression leading to a wide variety of outcomes including cellular or tissue remodelling, adhesion, migration, inflammation, secretion of various molecules, proliferation and potentially even apoptosis. The purpose of these responses is to achieve cell or tissue survival, homeostasis and the communication of the stress condition to other parts of the organism.
While certain types of tissue need shear stress to function correctly (like endothelial cells), others (the majority) rarely experience it. In the human body, most cells are organised in tissues and unless in direct contact with the blood or respiratory systems, they do not normally experience FSS. Thus logically cell types that experience FSS in vivo should be exposed to it in vitro while cells that do not, shouldn’t.
What is fluid flow shear stress?
Fluid flow shear stress is the force felt by cells when a liquid flows over their surface. The strength of the force will depend on the speed of flow and on the viscosity of the liquid. FSS can be constant (a more or less steady flow as seen as the blood flow through various tissues), pulsatile (periods of increased stress similar to that experienced by capillaries or alveoli) or oscillatory (periodic positive and negative stress (i.e. pressure as seen in endothelial cells within the heart)). Shear forces are usually measured in Pascals or Dynes per cm2 (1 Pa = 10 dyne/cm2) and are denoted by the greek letter τ (Tau).
Cells have sensors for FSS
Shear stress is detected by specialized proteins on their surfaces that act as sensors. One well-known family is the integrins. These are transmembrane receptors that link the extracellular matrix to the cytoskeleton and transmit signals bidirectionally across the cell membrane. The exact threshold for integrin activation by shear stress can vary based on cell type and experimental conditions but typically ranges from 0.1 – 1 Pa.
Additionally, there are mechanosensitive ion channels (like Piezo and TRP (Transient Receptor Potential)) and other membrane proteins which sense FSS. Activation thresholds for these channels also vary, with values in the range of 0.1 – 2 Pa.
Signalling pathways are activated by FSS sensors
When shear stress is detected, it triggers a cascade of intracellular signalling events. One critical pathway involves the activation of various kinases, such as focal adhesion kinase (FAK) and mitogen-activated protein kinases (MAPK). Activated by integrin signalling, FAK responds to shear stress. Mitogen-Activated Protein Kinases (MAPK): ERK1/2, JNK, and p38 MAPK can be activated by shear stress directly (range 0.2 – 1.5 Pa).
The activation of signalling pathways leads to changes in gene expression. Transcription factors, such as AP-1 and NF-κB, are often involved in shear stress-induced gene regulation and become activated. The expression of genes related to cell adhesion, migration, inflammation, and cytoskeletal remodelling is commonly altered.
Changes in gene expression lead to protein expression changes. For example, the shear stress induced upregulation of nitric oxide synthase (eNOS) in endothelial cells which leads to an increase in nitric oxide production (NO), and this plays a crucial role in vasodilation and maintenance of vascular homeostasis.
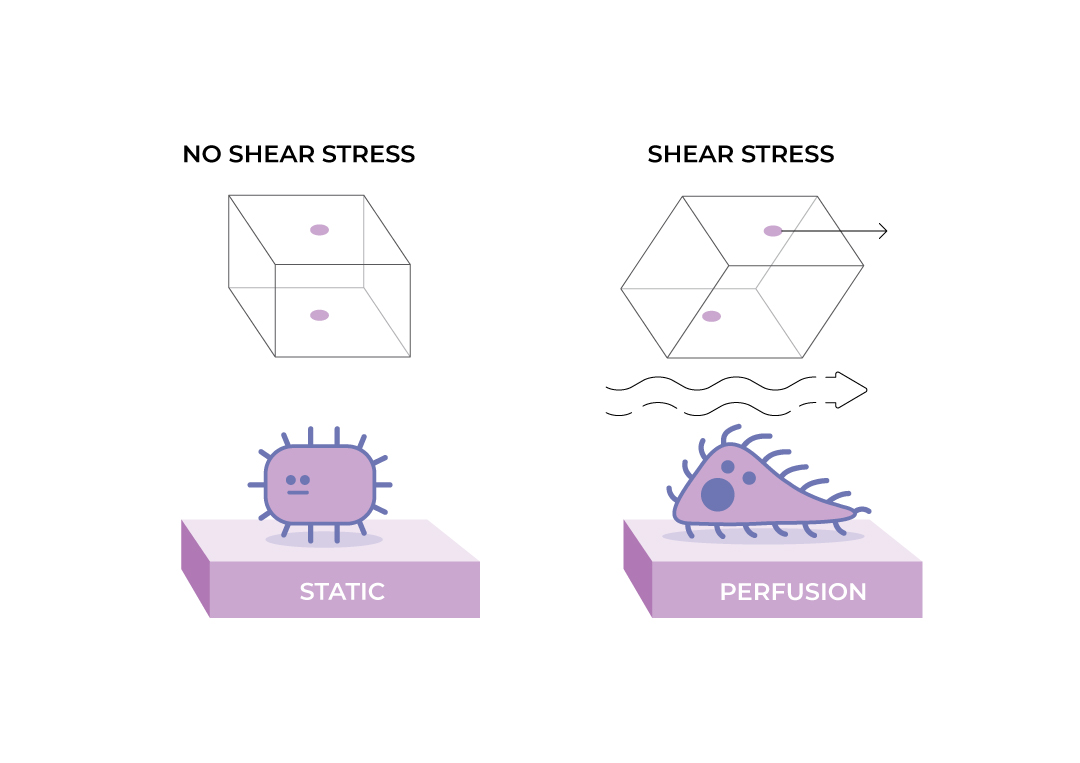
FSS and 3D cell culture
Out of the necessity to shorten the diffusion gradient, FSS is a common feature of many types of 3D cell culture. If the cells are not accustomed to FSS, then this will have adverse effects.
Cells can adapt to prolonged or repetitive shear stress through mechanisms like desensitization. This involves changes in the expression of certain mechanosensors or downstream signalling components – undesirable changes if understanding normal cellular metabolism or response to stimulation is the goal. Therefore, if not the intended subject of study, these responses will confound investigations of other aspects of cellular physiology and should thus be avoided.
A critical or lethal FSS for cells in culture is in the range of 0.3–1.7 Pascal (depending on the mammalian cell type).
In some of the equipment used for 3D cell culture, there is a magnetic stirrer in the bottom of the flask (or the flask itself is rotated). This significantly reduces the length of the diffusion gradients and keeps the constructs suspended in the media but results in significant FSS. For example, stirring a culture at a speed of 100–200 rpm results in a FSS of 0.3–0.66 Pa. Similarly, orbital shakers which rotate the culture flasks exocentrically at speeds of 20 – 60 rpm, result in FSS of 0.6–1.6 Pa). In this type of equipment, the FSS is so high that cell constructs are torn apart, resulting in a highly heterogeneous size distribution.
Cell constructs can also be cultured in bags which are gently agitated on a rocking platform. Set at an oscillation of 7 degrees at 20 rpm these induce unevenly distributed shear stress forces varying periodically between 0.01 and 0.6 Pa.
Microfluidic devices (also known as lab on a chip) have recently become very popular for cultivating cells because of the many advantages that they possess (low volume, easy assay, microtiter plate or microscope-slide format). It is possible in most of them to either perfuse the growth medium using pumps or to induce a gentle flow of the media by rocking the plate/slide. These also induce very low shear forces of for example 0.02–0.064 Pa when flow rates of 650 µL/min are used. Unfortunately, their miniaturised size perpetuates the adhesion induced tensile stress (caused by cell-plastic contact) and also precludes the formation of large multilayer 3D constructs which are essential for the recovery of in vivo functionality.
Finally it is possible to cultivate cell constructs in flasks that rotate about a horizontal axle (i.e. the clinostat principle). By adjusting the rotation speed to the size and shape of the constructs, it is possible to achieve the lowest shear forces seen in any cell culture equipment of about 0.01 Pa (at 20 rpm). This is below the sensor activation thresholds (of 0.1 – 1 Pa) and thus does not influence the cells’ gene expression. These minimal FSS forces allow the constructs to reach highly uniform sizes which in turn result in a homogeneous response when treated.
Without any flow, the cells in the centre of 3D constructs composed of about 5,000 cells are subjected to hypoxic/anoxic conditions. Cultivation in the ClinoReactor allows these spheroids to contain more than 80,000 cells before they reach the same hypoxic/anoxic conditions. This clearly shows that the device significantly increases the oxygen supply to the constructs without exposing them to FSS. In addition, the increased oxygen supply allows the constructs to be cultured for very long times (greater that 300 days) and reach large sizes which allows them to recover an in vivo mimetic phenotype.
A clinostat bioreactor (like the ClinoReactor) thus provides and thus is the best model for solid tissues.
Summary
In summary, shear stress has profound effects on cells in culture, influencing gene and protein expression through intricate signalling pathways. Understanding when to expose cells to shear forces and when not to, is crucial not only for basic cell biology but also for applications in tissue engineering, regenerative medicine, and the development of therapeutic interventions for conditions influenced by mechanical forces.
Connecting Your Research to Reality
Learn more about how you can benefit from using the ClinoStar 2 and clinostat principle when culturing cells in your lab.
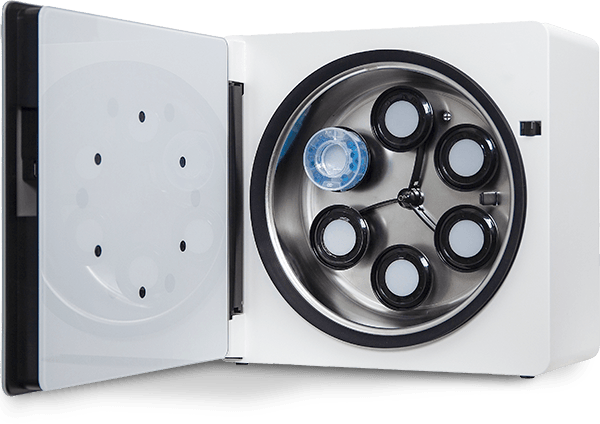